Pharmaceutical companies should leverage the unused potential of AI and big data to boost their sales management
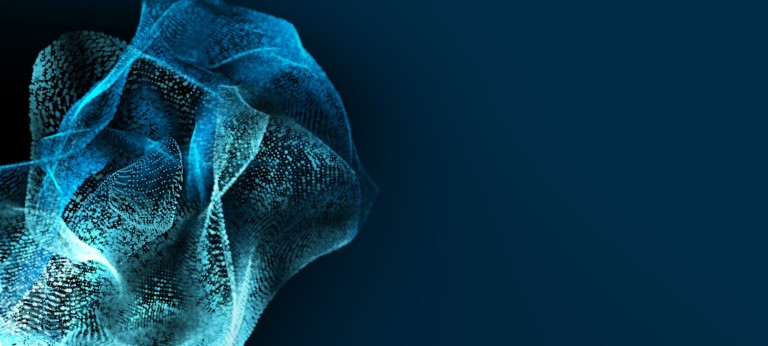
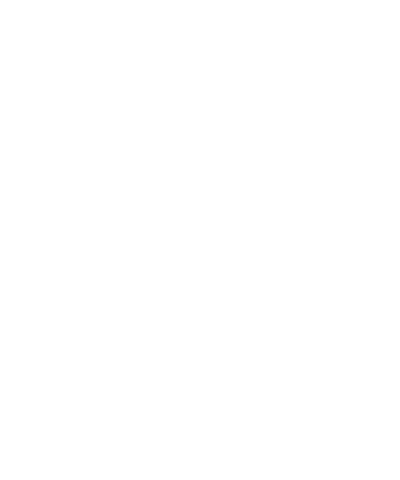
Cutting the cost of gene therapy manufacturing
By Morris Hosseini and Stephan Fath
Challenges and promising technologies
The recent introduction of gene therapies marks one of the biggest developments in the pharmaceutical industry in a generation. The therapies, which modify defective genetic material in patients with genetic disorders, are like no other pharmaceutical product and have the potential to treat or cure a multitude of diseases. Several have already proved to be highly effective: Luxturna has restored sight in some patients, for example.
However, the hope offered by these new treatments comes at a cost. Therapies can cost as much as USD3.5 million a dose, as is the case for Hemgenix, a one-off infusion to treat hemophila B. Manufacturing is the main cost driver. Processes for making gene therapies share no similarities with modern manufacturing methods for traditional pharmaceuticals, necessitating entirely new modalities. These are at an early development stage with none of the efficiency savings of mass production. In addition, production speeds are low. But as the technology exists, there’s a good reason to exploit it and drive down costs and time to market.
In this article we provide a brief market overview of the gene therapy sector, assess the challenges posed by gene therapy manufacturing and look at promising new technologies that may help to overcome them.
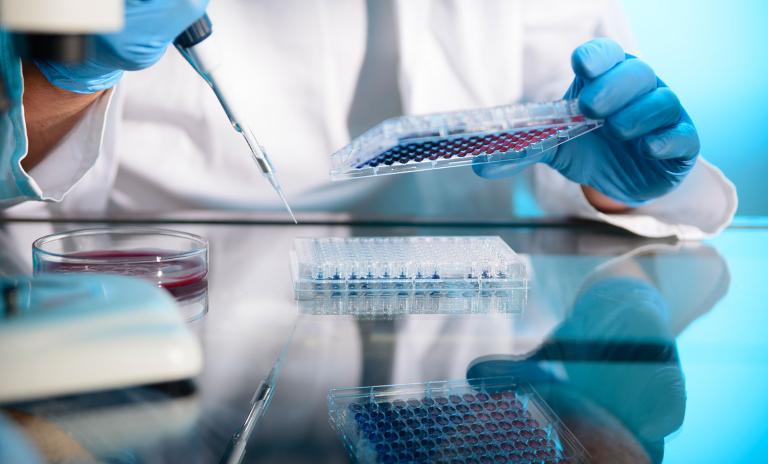
A lucrative opportunity – with a catch
Gene therapies have opened up a brand new – and highly lucrative – front in pharmaceutical sales. While only 16 products had received marketing authorization in the EU and 13 in the US since 2016, they have generated massive revenues. For example, Zolgensma, a treatment for spinal muscular atrophy made by Novartis, generated sales of more than USD3.6 billion between its launch in 2019 and 2022. Sales of non-cancer gene therapies, which make up a major share of the treatments available, are forecast to hit USD4.2 billion alone in 2028.
"Gene therapies have come a long way and we see enormous technological progress in terms of vector types but also manufacturing capabilities."
Despite this healthy outlook, the cost of manufacturing gene therapies is a major concern. Production and scale-up capabilities remain in their early stages, with laborious methods still required to produce one of the two main components of gene therapy, vectors, the vehicle that delivers the therapeutic cargo (usually DNA) to the cell. There is no one-size-fits-all vector and each has its own manufacturing process. Speed is another concern. It is important to rapidly produce novel therapies for trials in order to get to market quickly, but it takes time to produce them. So far, there are no established standards, for both regulatory and manufacturing practices, that can be used as a guide as the landscape is still evolving. As such, reproducibility is made difficult by the specificity of the cell line used in the upscaling from clinical to commercial. In addition, technology is advancing faster than manufacturers can adapt, meaning they are always playing catch up when it comes to manufacturing strategy. Investment levels are also falling. Below we look at these challenges in more detail.
Key challenges facing gene therapy manufacturing
Vector manufacturing
Vectors are the main driver of gene therapy manufacturing costs. Actual costs vary significantly depending on the type or subtype of vector required. There can easily be a 10-fold difference in yield between subtypes, for example. Optimal yields can only be achieved once significant expertise is gained in the manufacturing process, parent vector and specific subtype. No matter what the type, inefficiencies mean that not all yielded vectors carry a cargo.
Poor yields and inconsistencies remain a real problem. Yields between batches can vary greatly, while contamination can affect multiple batches. Inconsistencies are sometimes not detected until after fill and finish, resulting in the discarding of entire batches (worth USD2,000 to USD15 million depending on batch size).
Making bigger batches is another hurdle. Maximum sizes vary, and depend on the vector technology and expression system chosen. For example, in the case of adeno-associated virus (AAV) vectors, the most commonly used type, batch sizes are often limited to 500L as the addition of cargo becomes inefficient at higher volumes. As batch size has a direct impact on raw material spend per yield of vector produced (4 x 500L batches require more raw materials than a 1 x 2,000L batch), this results in higher raw material costs. In addition, there are higher quality control costs, as well as a depleted yield, compared to a single larger batch. Some players have, however, managed to achieve sufficient yields at volumes up to 2,000L.
The key challenge in upscaling is the maintenance of a homogenous mixture without damaging the vector-producing cells (you can only stir the pot so fast before the bubbles burst). Maintaining a homogenous mixture is essential for even transfection across cells, as well as nutrient distribution for growth and vector production.
Dosing also presents a cost challenge. A therapy’s indication and target organ play a key role in determining dosage, with some multi-organ indications requiring 100-times more doses than those of, say, ocular diseases. For example, in the case of Hemgenix, the one-off hemophilia B treatment, our calculations suggest that 500L batches produce enough units of therapeutic gene and vector to treat 2-3 adult patients. At an estimated cost of USD3-7 million per batch, this means the vector manufacturing cost alone of one dose could be in the range of USD1-2 million.
Manufacturing strategy
High manufacturing costs and the recent global economic pressures mean it is now more critical than ever to have a clear and realizable manufacturing strategy. As outlined above, vector manufacturing should be the main focus of the strategy as it presents the biggest hurdles (manufacturing of cargo is based on long-established industry standards).
Currently, around 70-80% of vector manufacturing is outsourced at early stages of development, and 45-50% is outsourced during late-phase and commercial-scale stages. This leaves specialized contract manufacturers limited to small-scale production.
Choice of strategy depends on several factors, with vector selection having a significant impact. As well as ease of manufacture, these include: whether the application of the gene therapy is done in vivo or ex vivo (some vectors are better suited to ex vivo settings); size of cargo (different vectors have different capacities); target tissue/cells (different vectors have different targets); gene delivery mechanism (these vary by vector); and immunogenicity (viral vectors can trigger dangerous immune responses).
"Manufacturing of gene therapies and especially vectors has become a critical factor, deciding about both the commercial and clinical success of new therapeutics."
Funding challenges
A recent global downturn in biotech funding has beset the gene therapy industry. Biotech venture capital funding fell by 45% between 2021 and 2022.
The main reason for this was that concerns about return on investment led to a stronger focus on cost of goods sold (manufacturing is the main driver of COGS in gene therapy). With an increasing number of globally marketed gene therapies, reimbursement negotiations are becoming more complex. For example, Novartis received marketing authorization for Luxturna, which is used to treat a rare retinal degeneration disorder, in the EU in 2018. But due to challenging reimbursement negotiations, it delayed Luxturna’s launch until it was granted US market authorization in 2022.
Trends and technologies in manufacturing
Fortunately, the gene therapy industry has not stood still in the face of these challenges. New trends and technologies are emerging in the two key areas of vector manufacturing and vectors themselves.
Vector manufacturing trends
Several new developments are helping to improve yield and batch size/consistency:
Specialization: A focus on a single vector type allows manufacturers to optimize the expression system and machinery required for production. This increases process development rates, decreases lead times, and reduces the risk/rates of contamination.
NextGen manufacturing: Advanced practices range from new bioreactor technology that optimizes cell density, to new formulations of feed stocks for batch maintenance to optimize yields. Due to lack of standardization and variation in manufacturing platforms, next-gen manufacturing practices greatly vary between manufacturers
Automation: High-throughput screening allows new vectors and subtypes to be tested under various conditions, helping to optimize yield with minimal raw material input. Also, automated cell culture maintenance can ensure greater batch consistency, and automated purification is scalable and can save time. Robotics may also have a place in fill and finish.
Downstream processing: The goal here is increased purity and concentration. Several chemical and physical techniques, such as new forms of capture chromatography and ultracentrifugation, are being tested, but these are specific to vector type and require fine-tuning and adapting to the manufacturing system.
Analytics: Regulations are becoming a major hurdle to commercialization, as evidenced by the recent delays in US Food and Drug Administration approvals for a Duchenne muscular dystrophy gene therapy called delandistrogene moxeparvovec, developed by Sarepta. Producing standardized data output across manufacturers with reproducible results at very high resolution is now more important than ever to streamline the path to commercialization. The challenge is to obtain this data with minimal sample sizes, as there are multiple testing/quality control stages throughout the manufacturing process. Currently sampling uses about 20% of each batch.
Vector trends
The main vectors
Five viral vectors are currently used in gene therapy. AAV have the biggest market share (45%), followed by lentiviruses (LV, 20%), adenoviruses (AV, 16%), herpes simplex viruses (HSV, 8% market share) and retroviruses (RV, 2%).
AAVs dominate for several reasons. First, they offer tropism to specific tissues, allowing cell targeting for both replicating and non-replicating cells. This tropism is being optimized by vector modification and hybridization of existing serotypes. Second, they have an effective gene delivery mechanism that does not insert into the chromosome, minimizing unwanted effects. AAVs also have limited immunogenicity due to their non-pathogenic background. Their main drawback is cargo size, which is limited to smaller DNA or RNA pieces.
The use of lentiviruses is also growing, spurred by their increasing potential for in vivo applications. They have larger cargo capacities than AAVs (more than 50% larger in some cases) and low immunogenicity. Also, their mechanism allows for stable application and they can expand tropism through genetic engineering and pseudotyping. However, there are concerns about oncogenesis.
Use of AAVs and LVs is likely to continue to grow, with market shares of 40-50% and 25-35%, respectively, estimated in five years’ time.
Non-viral vector technologies
New non-viral vector technologies are expected to be significant drivers of gene therapy manufacturing growth. Three main types exist:
Lipid nano particles (LNPs): LNPs are small vesicles made from synthetically produced fats, best known for their use as the vector in the BioNTech/Pfizer COVID vaccine. They are highly efficient at crossing barriers in the body, and relatively cheap to produce. However, their synthetic nature limits specificity and raises concerns about potential toxicity.
Polymeric nanoparticles (PNPs): PNPs have similar characteristics to LNPs, but can be manufactured from a broader range of molecules. This increased choice allows for greater cell specificity and overall functionality. Research into PNPs is still in the discovery/preclinical stage, but, due to their similarity to LNPs and potential for other industrial applications, they are expected to grow at a similar rate and be a major competitor. However, concerns also exist about potential toxicity.
Exosomes: Extracellular vesicles produced by mammalian cells, exosomes can be used as vectors themselves or as an additional vector coating for increased transport function and immune evasion. Their manufacture can be based on existing viral vector processes. While they possess less than a 1% market share, exosomes are a growing technology due to their broad use base.
Where Roland Berger can help
It’s clear that there is significant potential for improvements in gene therapy manufacturing, both in terms of cost and efficiencies. However, with the sector still in its infancy and rapidly developing, it’s unclear where savings will be made and which trends and technologies will win out.
Understanding current challenges and future trends in gene therapy manufacturing will be key to keeping COGS down, driving sustainable pricing and quickly getting novel gene therapies to trials and market. Roland Berger’s expert Pharma & Healthcare team has a thorough understanding of current trends in the gene therapy space, and is ideally placed to help large pharma companies and CDMOs build and develop their gene therapy platforms.
So please feel free to give us a call on any of the aspects covered in this piece, or on gene therapies more widely. We look forward to talking with you.